Centrifugal partition chromatography (CPC), also known as counter current chromatography, systems are preparative, pilot-, and industrial-scale liquid–liquid partition chromatography systems for the purification of various molecules and solutions. CPC uses two non-miscible liquid phases rather than a solid stationary support to prompt the separation of analytes in a sample. Analogous to a preparative high performance liquid chromatography (HPLC) column or flash cartridge, CPC systems require a prep-scale pump for the solvents, an injector to load sample, and optional system add-ons including a detector to observe the chromatogram and the fraction collector on the backend for collection of the separated component(s).
What follows is an overview of how CPC systems work and how they provide an automated purification solution for isolating compounds from natural product crude extracts when paired with compact chromatography systems.
CPC System Overview
A basic diagram of a CPC system (Figure 1) displays the rotor in the center held on a horizontal axis to allow for rotation. At each end of this axis are two rotary seals. Connections shown on the right indicate solvent flow from the pump onto the CPC column for separation and then from the CPC column to the detector and fraction collector.
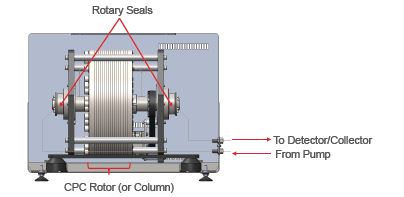
Figure 1: CPC System
The CPC column (Figure 2) is essentially a high-pressure resistance rotating chromatographic column. The rotor is composed of individual disks, each disk containing several twin cells linked by channels. The specific design of these twin cells provides for better retention of the stationary phase on the CPC column during the run while allowing for the use of higher flow rates. A higher flow rate provides faster separation resulting in higher productivity.
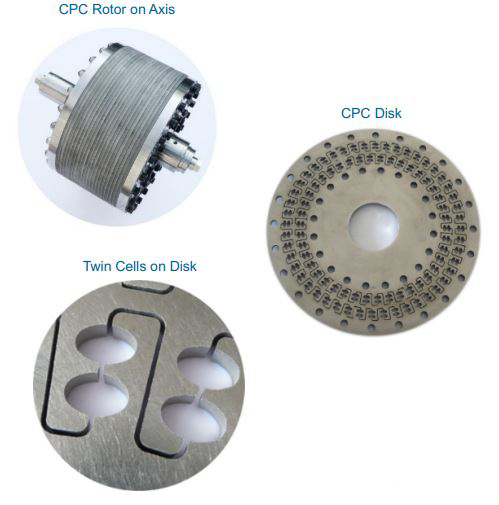
Figure 2: CPC Column
CPC General Principle
Consider each of the twin cells in the rotor disk as an individual separatory funnel. CPC employs a solvent system of two immiscible liquid phases created by mixing two or more solvents. The stationary phase is maintained by a centrifugal force and the mobile phase is pumped through the liquid stationary phase. The example diagram in Figure 3 illustrates a typical twin cell containing butanol and water. Butanol is the lighter upper mobile phase, while water is the heavier lower stationary phase.
Molecules A (KD = 1), B (KD < 1), and C (KD > 1) are added to the solvent system, mixed, and the two phases separate out to show the distribution of molecules between the two phases based on the molecules’ partition coefficients (KD). The molecules with a KD, close to a value of 1, are evenly distributed between the two phases which is molecule A, while the molecules with a KD <1 are mainly retained in the upper mobile phase represented by molecule B. The molecules with a KD >1 are more so retained in the lower stationary phase (molecule C). Once the separation takes place in one twin cell, the upper mobile phase is moved to the next cell in the column allowing an additional separation to take place. This process will occur hundreds of times.
Much of what occurs within the column is the result of volumes and flow rates. If molecule B has a KD of 0, molecule B will elute after one volume of the mobile phase is pumped through the CPC system. The flow rate will determine when it will elute. Therefore, if molecule A is truly KD = 1, it will come off following the volume of mobile phase inside the column, plus one column volume of stationary phase pumped through the system. With a KD of 2, molecule C will come off the column after a volume of mobile phase plus two column volumes. This is the scenario when run parameters have been fully optimized.
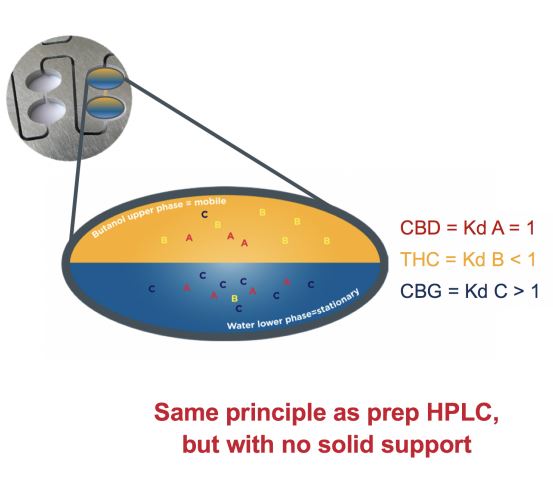
Figure 3: CPC general principle
Solvent System Determination
Choosing the solvent system in CPC is basically the same as selecting the column and eluent in HPLC. Depending on the end goal, it should be decided whether to operate the CPC in ascending or descending mode. Ascending is akin to normal phase, whereas descending is like reverse phase. This flexibility provides more options regarding solvent system selection.
When selecting a solvent system to use, the solvent system polarity must match the polarity of the molecules to be separated. Next, find the solvent system in which the partition coefficient for the compound of interest is 0.5–3. Note that if KD is too large, a long run time and a broad peak will result. A long run time increases solvent consumption and reduces productivity. If the KD is too small, coelution with other compounds is likely. The third objective when determining the solvent system is recognizing that the KD of the target compound must be different from other molecules’ KD, thus avoiding coelution. Compounds having the same partition coefficient will pass through the column in a similar manner, resulting in no separation.
Once potential solvent systems are identified, the “shake flask” method can be performed to see if they are suitable for CPC analysis. For the “shake flask” method, first create the solvent system by mixing together the appropriate proportions of each solvent. Next, transfer a volume of both the upper and lower phases to a different vessel. Then, add the crude extract to the vessel and mix. Once cantation is complete, there will be partitioning of the molecules within the crude extract amongst the two phases. Finally, analyze the upper phase and the lower phase (typically with thin-layer chromatography [TLC] or HPLC equipment).
The partition coefficient for each molecule can then be determined within the crude for this particular solvent system tested by a direct comparison of the concentration of each spot on TLC in the upper phase and lower phase. This is followed by comparing the percent area of each peak in HPLC in the upper or lower phase.
Steps of a CPC Purification Run
Once a solvent system is determined, a CPC run consists of six main steps, which can be scaled up to a larger production-scale unit:
- Choose descending or ascending mode and program the mode into the method so an automatic valve can switch to accommodate the setting.
- Load the CPC system with the stationary phase.
- Equilibrate the column with the mobile phase to determine the operating pressure of the current system as well as the retention of the stationary phase with current run parameters.
- Perform a sample injection.
- Assuming that a good operating pressure and good retention of stationary phase during the equilibration step is obtained, elute using the same conditions as equilibration. During elution, primary fraction collection will occur.
- Extrusion is performed and 100% stationary phase will be pumped back onto the column. This extrusion step accomplishes replenishing the fresh stationary phase on the column while also allowing for fraction collection of molecules heavily retained in the stationary phase.
Once the methodology is optimized, CPC runs can be performed in an elution extrusion mode. Following the initial loading of stationary phase and equilibration at the beginning of the day, load the sample, elute, and extrude, and then repeat. This can be accomplished because the fresh stationary phase is loaded on that CPC column during the extrusion step.
Effects of Flow Rate and Rotation Speed on Retention, Resolution, and Pressure
Figure 4 illustrates that retention of the stationary phase will decrease with increased flow rate; however, retention will increase with an increased rotation speed. Once the solvent system is determined, the right balance between flow rate and rotation speed to ensure appropriate retention of that stationary phase on the column must be found.
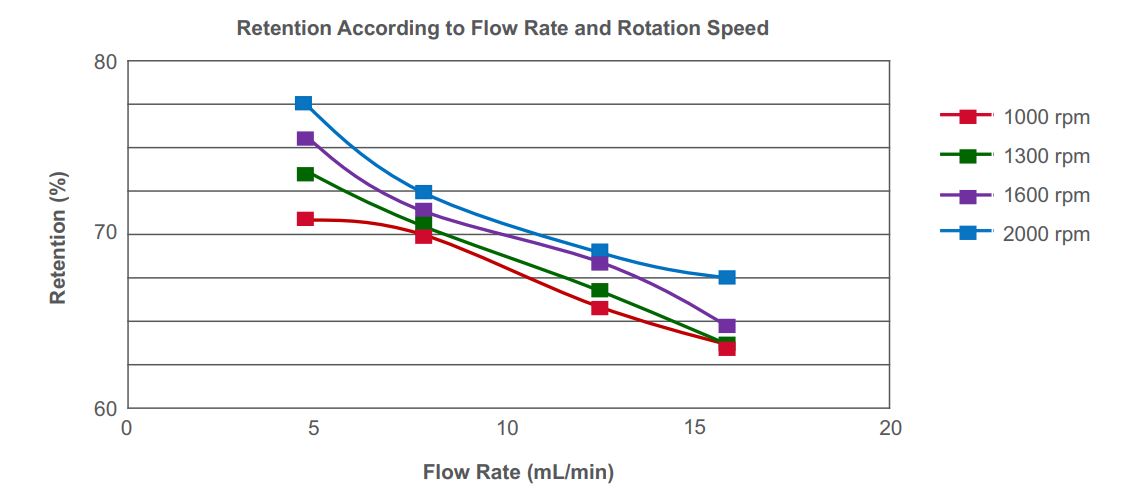
Figure 4. Effects of flow rate and rpm on retention
Resolution increases with rotation speed, while resolution decreases with an increased flow rate (see Figure 5). Operating at too high a flow rate will cause loss of stationary phase, thus poor retention. An ideal separation cannot be achieved if attempting to operate the CPC with poor retention of the stationary phase.
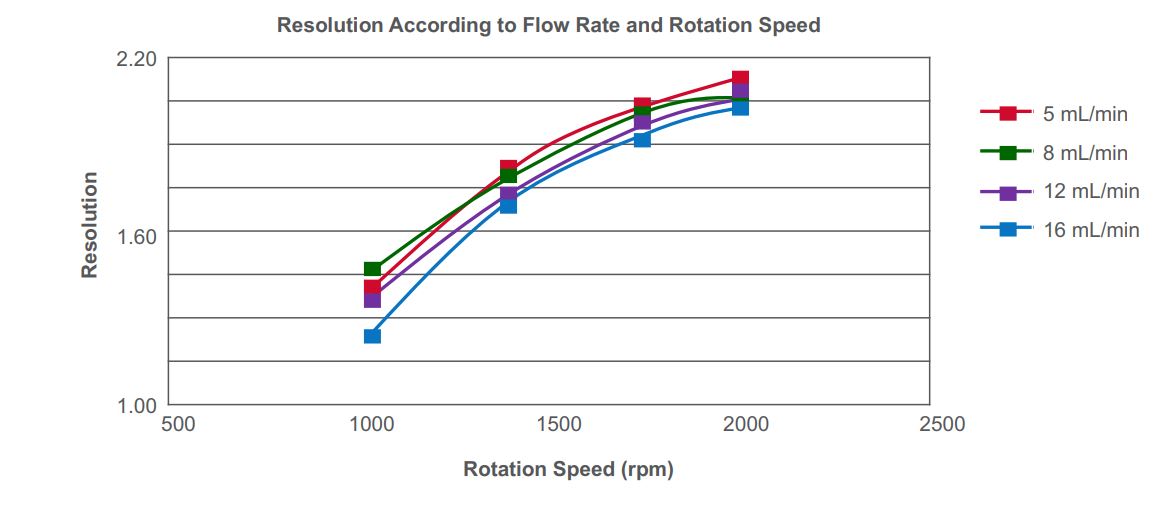
Figure 5. Effects of flow rate and rpm on resolution
As flow rate and rotation speed increase, pressure rises. This is one reason why the equilibration step is performed when developing methods. Changing the solvent system, flow rate, or rotation speed of the method, results in a change in the operating pressure of the system. Knowing the pressure at equilibrium is important when working toward optimizing the sample load capacity of that column.
Natural Product Applications
There are several possible applications for CPC systems within the natural products space, including purifying cannabinoids from cannabis oil, removing THC from cannabis oil, purifying omega-3 fatty acids from microalgae, and purifying steviosides from stevia.
An example comparing CPC and HPLC techniques for the purification of Caulerpenyne from seaweed (Caulerpa taxifolia) is shown in Figure 6. The HPLC chromatogram of the crude shows many impurities and additional purification by CPC analysis using Gilson’s CPC 250 and PLC 2250 Purification Systems results in the purified product. There is an increase in the isolated mass and yield, a reduction in solvent consumption, and a reduction in run time when the CPC is used as the purification method.*
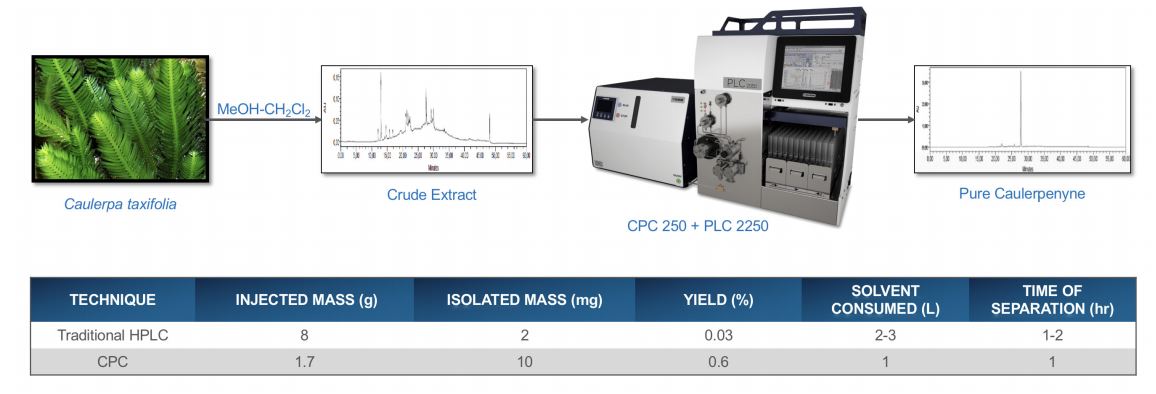
Figure 6. Natural product application: Caulerpenyne
Another example is a microalgae crude extract. During CPC purification, the fractions are collected, and those fractions are dried down to obtain purified products. Several groups of fractions obtained by CPC are pooled, and spotted by TLC, resulting in a yield of 23% with 99% pure EPA fractions, and a 10% yield with 98% pure myristic ethyl ether fractions.
Conclusion
Centrifugal partition chromatography is a cost-effective solution for natural product purification. Having no column to replace or silica to recycle, there is low solvent consumption when compared with traditional chromatography techniques, and no extensive pre-treatment of the sample is requested. The crude material is diluted, filtered, and loaded directly onto the CPC system with no initial clean-up methods being necessary. CPC systems allow for high injection capacities ranging from milligrams to multi-kilogram scale. The technique provides the capability to produce enriched extracts and pure molecules.
*This work was done in collaboration with the University of Nice and is published as “Caulerpenyne from Caul-erpa taxifolia: A comparative study between CPC and classical chromatographic techniques,” Phytochemis¬try Letters, Vol.20, 2017, p.406-409.
Gilson, the Right Choice for Natural Product Purification
Get the accuracy and precision you need for trusted results with VERITY® purification systems. Whether you isolate large or small molecules or need milligram to kilogram purifications, our VERITY systems offer you a wide array of components to build the perfect system to meet your specific needs.
CONTACT US